Eating a meal after a week-long starvation might be enough to make any meal seem hearty; but when a burmese python eats after starving for up to a whole year, the 'heartiness' quotient of the meal is raised to an entirely different level as the python's heart 'literally' grows by up to 40% after feeding. And, this is only one of many, quirky facts that can make the giant, scaly, unmoving, coiled hulk of a python seem interesting.
Unlike the many snake species that actively forage and hunt for their prey, the pythons (and some others like boas, vipers and pit-vipers) employ a sit-and-wait tactic for hunting. Although this saves them the energy costs of active hunting, it also limits their feeding opportunities and imposes additional adaptations as their body has to adapt to large intervals (up to a year for a burmese python) between meals. These meals, though rare, are not small by any means - a python is capable of consuming anywhere from 80-150% of its own body weight in one meal! That's like a 100 lb. person eating anywhere from 80-150 lb. of food in one sitting. These dietary extremes have resulted in unique physiological adaptations.
As a python lies in wait for its next meal, it enters a stage of quiescence and down-regulates both the structure and function of its gut. At the risk of damaging its own lining in the absence of food, the stomach stops producing any acid. The pancreas and the gall bladder stop their secretions and the intestinal epithelium (which normally absorbs the nutrients from a meal) atrophies. The proteins that transport nutrients across the gut lining are also shut down. In fact, in addition to the gut, other organs of a starving python such as the liver, heart, pancreas and the kidneys are also dramatically shrunk and inactive during starvation.
But things change dramatically with that one meal.
When a cavalier prey passes by an inert python, it is often unprepared for the viciousness of the attack and the crushing defeat that are to follow. The python lunges on its prey, impaling it with its teeth and then gradually crushing it to death by coiling around it. The muscular hulk of the python coils and crushes its thoracic cavity suffocating it to death. Once the prey is dead, then begins the slow process of swallowing it, usually head-first. The python uses its paired set of pterygoid teeth (on the palate) in alternating steps and literally walks over the prey's skull internalizing it in the process. Considering the enormous sizes of a python meal, its skull is extremely flexible and mobile with multi-hinged jaw joints and a flexible ligament between the lower jaws. The process of swallowing a large prey is further aided by the axial muscles (lining the body wall) that gently propel the food - first into the expandable esophagus and then into the waiting stomach.
Although fascinating in itself, the ingestion of the prey is only the beginning of the wondrous world of a python's alimentary canal. The prey begins to putrefy naturally soon after ingestion and generates large amounts of gas (that luckily we don't have to smell) and this further increases the girth of the python. Before you conceive of an exploding python, let me clarify that this build-up of gases is not enough to cause an explosion but it does cause severe compression and exert additional pressure on the internal organs. It also interferes with ventilation and blood flow (much more than how one might feel after two thanksgiving meals). Thus, the python needs to digest the large and intact prey before it starts decaying significantly and the only tool at its disposal is a dormant gut with no secretions.
A Burmese python swallowing a laboratory rat which it had killed by constriction. (B) A Burmese python 24 h after consuming a rat meal greater than 50% of the snakeʼs body mass. This snake had experienced further distension of its body after feeding due to the build up of gases within the ingested dead rat.
Unlike most mammals including us, the starving python does not have baseline acid levels in its stomach. Remarkably though, within a day of the meal, the quiescent gastric lining is rapidly re-activated and it starts pumping massive amounts of H+ and Cl- ions into the stomach lumen dropping its pH from being neutral 7 at rest to extremely acidic (at pH 2) within almost 24 hours of the meal.
The post-feeding profile of gastric pH for Burmese pythons demonstrating the rapid drop in pH after feeding, the steadymaintenance of a very acidic pH during digestion, and the rise in pH upon the completion of gastric digestion when acid production ceases.
The stomach also releases an inactive protease enzyme, pepsinogen, which gets activated under the acidic conditions. The cleaved and now functional pepsinogen acts on the prey to dissolve the soft tissues and skeletal elements. By the third day, only 25% of the ingested meal (which could be as big as a kangaroo) remains within the stomach and it largely consists of the difficult-to-digest parts such as the trunk-vertebrae, the hind limbs, tail and hair. By about 6 days, the prey is almost completely dissolved and all that remains is a mat of hair to be sent on its way out.
The post-feeding profile of gastric pH for Burmese pythons demonstrating the rapid drop in pH after feeding, the steadymaintenance of a very acidic pH during digestion, and the rise in pH upon the completion of gastric digestion when acid production ceases.
The stomach also releases an inactive protease enzyme, pepsinogen, which gets activated under the acidic conditions. The cleaved and now functional pepsinogen acts on the prey to dissolve the soft tissues and skeletal elements. By the third day, only 25% of the ingested meal (which could be as big as a kangaroo) remains within the stomach and it largely consists of the difficult-to-digest parts such as the trunk-vertebrae, the hind limbs, tail and hair. By about 6 days, the prey is almost completely dissolved and all that remains is a mat of hair to be sent on its way out.
As the stomach acids and enzymes work, small amounts of the digested food are metered into the intestine by the opening and closing of the pyloric sphincter - a valve between the stomach and the intestine. On entering the intestine, the acidic pH of the stomach chyme is neutralized by the alkaline environment and the pH increases to 6.5 within a few centimeters. Other enzymes from the bile such as the amylases, lipase's and proteases from the pancreas also join the party as the food is broken down to its elements in the intestine.
Once the food has been digested, the next step is the absorption of the broken-down nutrients - normally done by the tiny finger-like projections (called villi) in the intestinal wall. The python's intestine and the villi, however, are completely shriveled during the starvation period to conserve energy. However, within a few hours of the feeding (while the prey is still intact in the stomach) the dormant intestine is woken up to a flurry of activity - all in anticipation of the nutrients that will be coming in. The intestine doubles the length of the villi, up regulates the amino acid uptake rates and the activity of its enzymes. In fact, within 24 hours of the feeding, the mass of the intestine increases by up to 70%.
Images of the small intestine of similar-sized Burmese pythons fasted and at 2 and 10 days post-feeding (DPF). By 2 DPF, the intestine has increased in diameter due primarily to hypertrophy of the epithelial cells; a response that has reversed by 10 DPF.
In keeping with the increased flurry of metabolic activity, there is also an increase in the size of most, if not all, internal organs of a python - the liver, the kidneys, the heart and the pancreas. In fact, in order to keep up with the increased need for energy, nutrient transport and organ growth, the python heart grows in mass by 40% within 48 - 72 hours of a large meal.
The growth of an organ can be achieved by two means - by either an increase in the number of cells that make up the organ (hyperplasia) or by an increase in the size of individual cells (hypertrophy). The growth is a python heart after a meal appears to be hypertrophic or driven by increase in cell size than number. In fact, studies show that a growing python heart up-regulates a system of genes akin to what happens when we augment our cardiac output - either due to pregnancy or with exercise. These events are rather different from the pathological program that is activated by hypertension, heart attacks etc. The quirky physiology of a python heart thus becomes an interesting subject to study even from the perspective of human health and disease.
The increase in the size of a python heart post-feeding is characterized by cellular hypertrophy. Compare the heart size of a starving python and that at 3 days post-feeding (DPF) Scale bar, 2 mm.
Two days after feeding, after absorption of the nutrients, the unabsorbed material (largely hair) begins to enter the large intestine. With each continuing day of digestion, the cecum and the large intestine fill with unabsorbed waste material, that is ultimately expunged as a bolus along with urate. Within a week after the meal, the last of the prey exits the stomach and the small intestine and these organs begin the shut-down process anew. Within ten days, the stomach pH rises, the enzyme activity drops down and the intestinal mass declines. The other organs too begin to shrink to their former state.
The question that remains though is what regulates this system level change in the organ size and function.
Studies suggest the involvement of two pathways - hormonal (systemic effect of hormones) and luminal (arising from the gut lumen itself) in mediating these rapid changes in organ size and function. The levels of many hormones that regulate gut function such as neurotensin, glucagon, insulin and cholecystokinin increase sharply after a meal. Experiments where small segments of the gut were surgically isolated from the rest of the gut but kept connected to the circulation were also able to increase their function upon a meal suggesting that a link to the blood circulation was sufficient to drive these systemwide changes. The gut lumen itself was also seen to play a role in triggering the initial responses as direct injection of nutrients (amino acids and proteins) into the python's small intestine was able to reawaken the intestine from dormancy. Interestingly though, injecting saline, glucose or lipids did not have the same effect on the python's intestine.
The factors underlying the increased heart size are however much less clear. In order to study the possible involvement of systemic factors on cardiac output, scientists injected blood plasma from fed and starving pythons into mice. Much to their surprise, components in the plasma of a fed python had similar effects on the heart of a starving python and of a mouse and caused significant increase in their respective heart sizes.
Fatty acids in the blood of a fed python induced cardiac growth in a starving python. Infusing fasted pythons with fed plasma or a mixture of the three individual fatty acids resulted in increased heart mass (heart weight/body weight) thus mimicking the effect of a meal (3 DPF). Bovine serum albumin (BSA) was used to solubilize FAs and is therefore used as control here to show that it alone did not have the safe effect as the fatty acids.
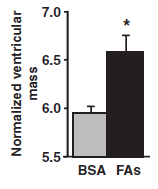
Seven-day infusion of the same three fatty acids in mice also resulted in increased heart growth (as measured by the size of the ventricle).
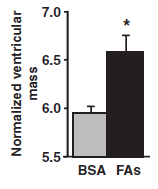
Seven-day infusion of the same three fatty acids in mice also resulted in increased heart growth (as measured by the size of the ventricle).
Interestingly, the fed-python-plasma continued to function in a starving python even after treatment with heat and a protease - suggesting that the active ingredient was likely not a protein. Scientists then suspected the involvement of lipids (since they are resistant to heat and proteases) since feeding led to sharp increases in the levels of lipids and fatty acids in the python blood (see the turbid opaqueness of python plasma after feeding). Careful studies identified three commonly known lipids - myristic, palimitic and palmitoleic acid to be responsible. Injecting these three lipids in the precise ratio to a starving python over a 7 day period resulted in significant growth in the heart - comparable to what would have been triggered by a good meal. Strangely however, the effect of these lipids was limited to the heart as the other organs - liver or muscle showed no change. Supplementing these lipids individually also had no effect.
Careful observation also shows that the levels of the lipids shoot up in the plasma even before the digestion has begun in earnest (see peaks at days 1 and 2 and compare with the X-rays of the prey from earlier). This suggests that the lipids are not coming from the digestion of the prey but are indeed a signal produced by the python to synchronize its various organ systems and to prepare them for the upcoming phase of hyperactivity.
Also of interest is the fact that despite such high levels of lipids in its blood (to the extent that the plasma appears cloudy), the python heart shows no lipid deposition. This again would be rather useful to many of us who worry about inching closer to a heart attack with every blob of butter or every additional french fry. If only, our hearts would be more like a python's and not deposit the fat around its vessels - we could eat everything that we wanted and yet avoid the consequences (or at least some of them).
Despite the creepy-crawlies that I had previously associated with a python, now, it is a fascinating creature with some extremely cool biology. Imagine someone emerging from a near comatose state only to successfully complete a marathon! That is the equivalent of what a python does every time it eats a meal.
References:
2) Digestive physiology of the Burmese python: broad regulation of integrated performance
by Stephen M. Secor The Journal of Experimental Biology 211, 3767-3774 in 2008